Researchers from the Australian National University (ANU) have discovered a way to create interlayer exciton pairs using bilayer semiconductor technology, paving the way for faster devices that consume less energy.
This research could lay the groundwork for a new generation of smartphones and computers and next-generation technologies that high-speed computing, information processing, and data communication require.
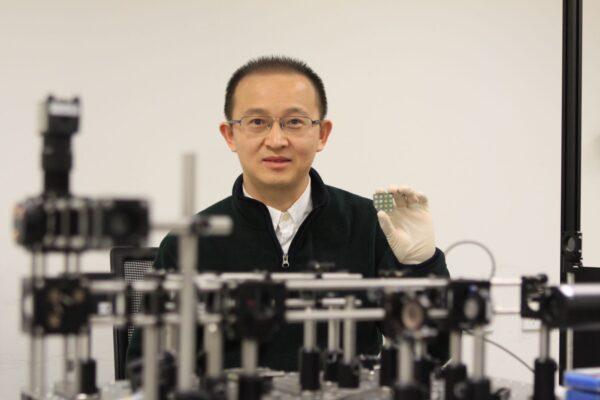
Structure of the Tech and How it Works
The technology is developed by sandwiching two sheets of bendable monolayer semiconductors together.“The double layer structure is made of two different semiconductors (two different materials),” said Lu in an email to The Epoch Times.
“In our case, the top layer is tungsten disulfide (WS2), and the bottom layer is tungsten diselenide (WSe2). Those two layers are both atomically thin, and they have different physical properties.”
The semiconductor bilayer allows for the creation of exciton pairs between the two layers when they absorb light. Excitons are quasiparticles that are formed by the attraction between a negatively charged electron and a positively charged hole, which is created when the absorption of light kicks out an electron.
“When a semiconductor absorbs light, an electron (negatively charged) can be kicked out (or excited) from the original location, leaving a ”hole“ (positively charged).”
Lu said that the excited electron and positively charged hole could bind together by way of attractive interaction to form an exciton.
“In our bi-layer structure, the excited electrons will sit in the top WS2 layer, and the ”holes“ will sit in the bottom WSe2 layer—this is determined by the physical properties of those two semiconductors.”
Driving Excitons with Voltage
Electrical current can be thought of as the flow of electrons; in circuits, the flow of electrons is driven by voltage— electrical pressure created by the electrical potential difference between terminals. The chemistry inside batteries, for example, causes electrons to move to one side, leaving an absence of charge on the other, creating a potential difference.Lu said that voltage can still be used across the circuit to drive the movement of interlayer excitons because the negatively charged electrons and positively charged “holes” sit in two different layers.
The electrons, although they have formed attractive interactions with the positively charged “holes” in the below layer, are still negatively charged within the top layer because the “holes” are absent from the top material. Thus, they can be pushed through the top layer of the transistor as electrons move through any conductive material when driven by voltage.
The bottom and top layers are connected by a conductor, and when an electron flows into this bottom layer, it can fill the space of a positively charged “hole.” The electrons flow through the bottom layer by moving into the positively charged holes and then emitting out of the layer and into the rest of the circuit when they are excited by light absorption.
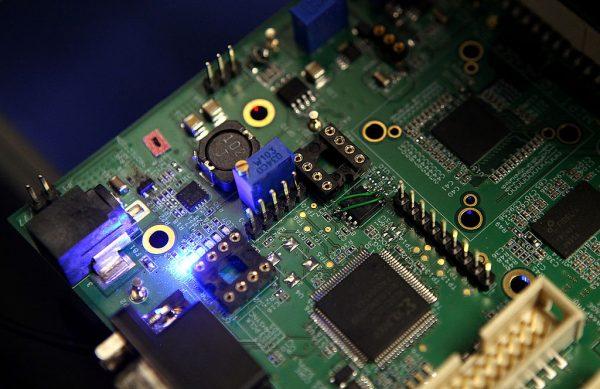
Next Step to New Generation Tech
The discovery also has the potential of helping researchers in achieving superfluidity, a condition where electrical currents can flow without resistance and, therefore, have no loss of movement energy, as collections of excitons condense into a superfluid.PhD researcher and first author on the paper, Xueqian Sun, said that superfluidity is best envisioned as a “superhighway” where excitons can travel at extremely high speeds like cars on a freeway.
“The current generation of semiconductor technology used in our smartphones and laptops limits the speed that excitons can travel, stopping them from reaching their full potential,” Sun said.
“A good way to visualise this is to think of a car that is bumper-to-bumper on a highway full of traffic. A car can only travel so fast in these conditions, and the same is true for excitons.”
Professor Lu said that in current semiconductor technology, superfluidity is hard to achieve, and the excitons can be scattered by many other particles, similar to a car moving in the city.
“In traditional bulk III-V semiconductors, the binding energy of excitons is very low (only a few meV), and those excitons can only survive at very low temperatures.”
“The new atomically thin semiconductor structure can host excitons with much higher binding energies, which allow them to survive at much higher temperatures (like room temperature),” he said.
“The interactions among those interlayer excitons in the new semiconductor structure are also highly enhanced, which would help achieve superfluidity.”
Prof. Lu said that the next goal is establishing a superhighway that functions at room temperature, as this is essential to integrating this semiconductor structure into smart devices.
“To be honest, I do not know how to do it at this moment,” he said. “I need to discuss this with physicists and potentially collaborate with them.”