The spike protein responsible for COVID-19 infection is like a loaded spring, with a catch, according to new research.
[embed]https://youtu.be/gVjbMMHv-TA[/embed]
The catch comes in the form of glycans, sugar molecules that are attached to the “head” domain of the spike and enclose it while the fusion peptide “tails” go in search of a cell to invade. The cage formed by these steric (nonbinding but influential) glycans hold the head domain in check, giving the fusion peptides time to look around.
Once the virus finds a host, the head and viral membrane are pulled toward the tail, fusing with the unfortunate cell.
“The glycans give the fusion peptides enough time to diffuse,” says co-principal investigator José Onuchic, co-director of the Rice University-based Center for Theoretical Biological Physics (CTBP). “Finding a way to speed up the spike’s conformation may be one approach to keeping the peptides from fishing for a host cell.”
While little can be done about the presence of glycans, revealing their significance in the spike’s mechanism could offer previously unknown opportunities to protect against COVID-19, says co-principal investigator Paul Whitford, an associate professor at Northeastern University and senior scientist at CTBP.
“Glycans are all over the place, and in principle, a glycan-free system would make the virus pretty ineffective—but introducing something that removes them in the cell would likely have major side effects,” he says. “However, the simulations show us the full conformational change of the spike, which basically has to turn inside out. This huge rearrangement reveals many more points where we could potentially target the system.
“Some of the elements are completely buried at the start and finish, but transiently, they’re accessible,” Whitford says.
A previous study involving the team, a collaboration with experimentalists at Rutgers University, used computational models to find hooks on the spike for an inhalable vaccine. The new study takes a more comprehensive look at how the spike changes to deliver its viral load to the cell.
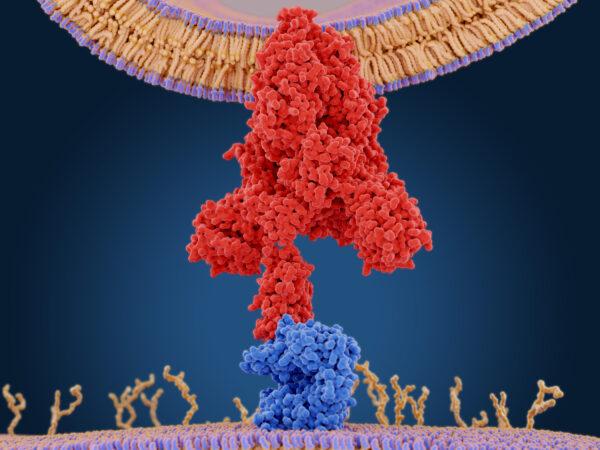
In the model, the process starts when the spike 1 (S1) domain that covers the spike 2 (S2) head finds a receptor known as ACE2, a protein commonly found on the surface of cells. S1 dissociates from the spike and releases the head, which climbs toward the viral capsid and is enveloped by glycans.
Once the probing peptides attach to a cell, the glycans release their hold on S2, which moves like a zipper along a connecting domain to bring the virus into contact with the cell.
That, in turn, should lead to further work by experimental labs and others who wish to build onto the simulation. Whitford notes all the models and data will be freely available to the research community.
“This has been a great experience,” Dodero-Rojas says. “We started this project during my first year of graduate school, and besides how challenging it has been amid the pandemic, the possibility that our work may help fight COVID makes it highly rewarding.”
“I’m optimistic about the next steps,” he says. “Potentially, the mechanism we show could be a target for new therapies or strategies to reduce SARS-CoV-2’s impact on humanity.”
The National Science Foundation, the Welch Foundation, the Northeastern University Discovery cluster and research computing staff, and the AMD COVID-19 HPC Fund program supported the research.